New variants of SARS-CoV2 have hampered our attempts to control the pandemic in recent month. The alpha variant, which emerged in the UK in late 2020, was a driving factor for a devastating wave of infections and high number of deaths in Europe at the beginning of 2021. While alpha was contained relatively fast, the currently dominant delta variant has massively changed the course of the pandemic.
Delta’s increased transmissibility and partial immune evasion has led to multiple waves with record infection numbers registered all over the world. The rapid development of multiple highly effective and safe vaccines, however, has helped to better control the virus. Vaccination campaigns especially in the developed world have brought down numbers of hospitalisation and deaths but infection rates are still high due to vaccine hesitancy in relatively large proportions of the population and a decreased protection against infection with the delta variant. The emergence of novel, more dangerous variants, which become less efficient to contain with our current vaccines, illustrate the need for an update of our vaccination approach in the future.
Corona variants deviate from the initial Wuhan strain
All approved vaccines were modelled based on the original virus strain first sequenced in Wuhan, China. New variants, however, have acquired structural changes of the proteins on the surface of the virus that have the potential to make our vaccines less effective. Our current vaccines protect exceptionally well against all currently circulating variants and next generation vaccines considering changes in the structure of virus proteins are already in development by leading pharmaceutical companies. However, predicting the evolutionary change of protein shapes in future variants is difficult to model if not impossible. A vaccine that protects against all future versions of the original virus would be a major step forward in our attempt to bring this pandemic to an end. Recent advances in science and technology might make this possible in the near future and even help us contain not only SARS-CoV2.
A pan-corona vaccine protecting against multiple virus strains
New technologies and a better understanding of our immune system as well as its interaction with corona viruses can be helpful to develop vaccines with more robust protection from virus variants taking into account the changes in the virus genetic information. However, researchers are even considering targeting not only SARS-CoV2, responsible for the ongoing pandemic, but also corona viruses more broadly, which have pestered humanity for a long time. While COVID-19 is by far the worst pandemic driven by corona viruses to this date, we must not forget that this is not the first epidemic caused by a corona virus.
SARS and MERS caused major outbreaks within the last 20 years, while being regionally contained, and it is very likely that SARS-CoV2 is not the last dangerous corona virus. In fact, increased deforestation and contact between humans and wild animals in developing countries make it more likely that another corona virus jumps the species barrier in the next decades with potential devastating consequences for societies. The development of a pan-coronavirus vaccine that protects us from multiple already known virus strains and future variants is therefore of uttermost importance to protect us not only from COVID-19 but also from future threats as well as other corona viruses in human circulation that cause only moderate cold-like symptoms.
Our current vaccine concepts aim to present a specific part of the SARS-CoV2 virus to our immune system. Most approaches have selected the spike protein due to its location on the surface of the virus and easy accessibility for immune cells and neutralising antibodies. However, all current vaccines use the same spike protein version isolated from the original Wuhan strain of the virus, which makes this approach susceptible for immune evasion if new variants acquire mutations that change the structure of the spike protein and reduce binding affinity of antibodies. Corona viruses show high conservation of certain domains of the spike protein that are necessary for virus entry into our cells.
Differences in protein structure encoded by the virus RNA are responsible for the emergence of different variants and virus families within the genera of corona viruses. Presenting multiple versions of the spike protein derived from different strains or families of the virus, however, allows the immune system to get to know multiple shapes of the spike protein and launch a broader immune reaction targeting multiple structures. This can be achieved by different methods depending on the vaccine platform used. For instance, mRNA or vector vaccines encode the genetic information of the spike protein as RNA or DNA respectively, which are read by our cells and produce the spike protein. By modifying the RNA or DNA sequence, multiple spike versions can be encoded. These different RNA or DNA versions can be combined and used in a single injection.
Plug and Display vaccine platforms
The mRNA and vector vaccines are the most common technologies used to induce protection against COVID-19 at the moment. However, further technologies are in development aiming to directly present the different protein versions to the immune system and showed great potential in animal models and clinical applications. Our immune system evolved to recognise viruses based on their shape and molecular structure. The proteins used to teach our immune system to recognise SARS-CoV2 are relatively small and injecting them directly in our arms is less effective as proteins alone do not resemble viruses very well and are less interesting for our immune system even in the presence of adjuvants provoking strong immune responses. mRNA and vector vaccines solve this problem by expressing these proteins on the surface of our own cells, which resembles an actual virus infected cell much more convincingly.
Additional strategies aim to construct virus-resembling nanoparticles that carry the proteins of interest on its surface but are non-infectious and do not contain any genetic information. The immune system is then tricked into experiencing a potentially dangerous virus infection and launches a strong immune response against the proteins presented on the nanoparticle surface. This technology has been successfully applied to immunise against the human papillomavirus (HPV), which causes cancer, as well as the Novavax vaccine against COVID-19, which showed promising clinical results and could get EMA/FDA approval soon (Shinde et al., 2021).
While these nanoparticle vaccines are safe and very effective in preventing infections for multiple diseases, they only protect against a specific virus variant. However, combining the nanoparticle strategy with multiple virus variant proteins allows the immune system to develop immunity against many virus strains simultaneously: The mosaic nanoparticle technology relies on large nanoparticles that resemble a virus like shell decorated with multiple versions of the virus spike protein, thereby mimicking a hybrid virus combining different versions of the immunogenic protein. The immune system can then directly recognise the foreign antigens and produce antibodies for a wide range of molecular structures.
A recent study published in Nature demonstrated the efficiency of these next generation vaccines in macaques (Saunders et al., 2021). The authors constructed a self-assembling nanoparticle consisting of a 24-subunit ferritin derived from Helicobacter pylori with an N-terminal sortase A acceptor sequence. These nanoparticles can bind proteins tagged with a sortase A donor sequence by conjugation through a sortase reaction thereby placing the tagged protein on the surface of the nanoparticle (Fig 1 A). Macaques injected three times over several weeks with nanoparticles conjugated to the receptor binding domain (RBD) of SARS-CoV2 (Fig 1 B) showed strong production of neutralising antibodies against the virus (Fig 1 C) and were highly effective in neutralising virus particles derived from variants that showed higher levels of immune escape. Notably, the levels of virus-neutralising antibodies were significantly higher compared to macaques injected with mRNA vaccines used in products such as the BioNTech or Modern vaccines. These findings show the benefits of nanoparticle-based vaccine technologies and highlight future ambitions to further increase the efficacy of COVID-19 immunisation.
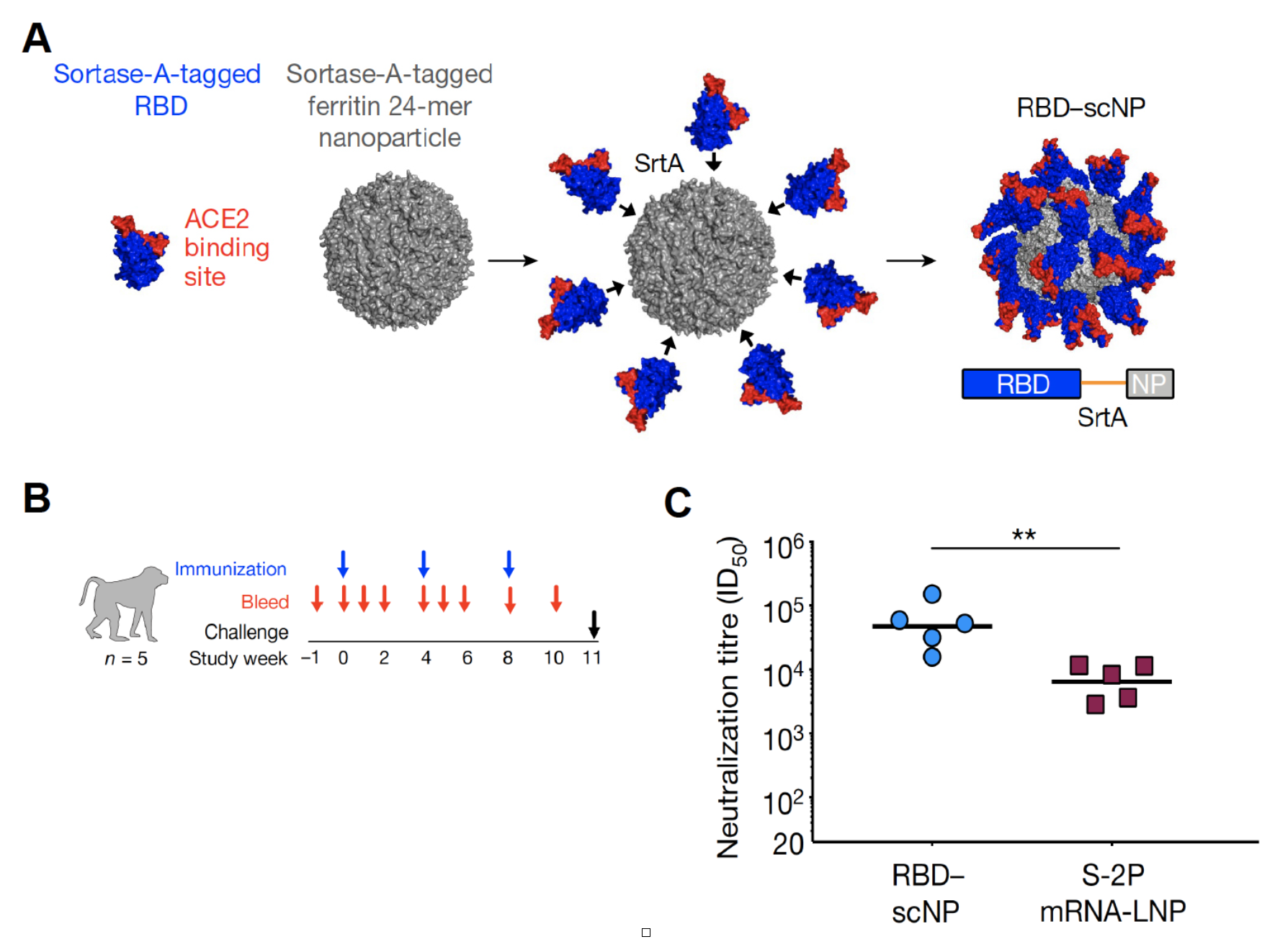
Figure 1:
RBD-nanoparticles elicit extremely high titres of SARS-CoV2-pseudovirus neutralizing antibodies
A) SARS-CoV-2 RBD (blue and red)–H. pylori ferritin (grey) nanoparticle sortase (SrtA) conjugation. Model and two-dimensional class average of negative-stain electron microscopy (EM) of the resultant RBD–scNP are shown. B) Design of the cynomolgus macaque immunogenicity and challenge study. C) Serum neutralization ID50 titres from macaques immunized twice with RBD–scNP (blue) or S-2P mRNA-LNP (burgundy). **P = 0.0079, two-tailed exact Wilcoxon test. n = 5 macaques. Modified from Saunders et al., 2021
The latest approach: Mosaic nanoparticle vaccines
Nanoparticles conjugated with only one version of the RBD were efficient to induce immunity against multiple strains of SARS-CoV2. However, they likely don’t protect well from different coronavirus families or future variants that might show higher immune escape. In order to develop a pan-corona vaccine the immune system has to be confronted with multiple virus-derived RBDs from different virus strains (Fig 2 A).
Researchers at Caltech and Oxford were able to construct a nanoparticle conjugated to multiple versions of the RDB of beta coronaviruses (Cohen et al., 2021). The team used a computationally designed virus-like particle (VLP), SpyCatcher003-mi3, which assembles into a nanoparticle that can be conjugated with multiple proteins on its surface (Bruun et al., 2018). The SpyCatcher protein is fused to the nanoparticle that can bind a small peptide sequence named SpyTag (Fig 2 B).
This system is derived from the Streptococcus pyogene FbaB protein by splitting and engineering the CnaB2 domain and allows the attachment of up to 60 individual proteins to the nanoparticle’s surface. The researchers conjugated the nanoparticle with multiple combinations of the RBD from several corona viruses followed by injection into mice (Fig 2 C).
By measuring the levels of virus-neutralising antibodies against multiple corona virus families produced by immunised mice, the researchers were able to evaluate the potential protection against those viruses. Mice injected with SARS-CoV2 soluble protein not conjugated to the nanoparticle showed low levels of neutralising antibodies against SARS-CoV2 and the original SARS virus as expected (Fig 2 D).
However, fusing the RBD of SARS-CoV2 to the SpyCatcher003-mi3 nanoparticle greatly increased the production of protective antibodies. Nanoparticles fused to different combinations of RBDs derived from multiple beta corona viruses was highly efficient in inducing the production of neutralising antibodies against multiple virus families. Most notably, combinations of fused RBDs not including the SARS-CoV2 RBD were still highly efficient to produce neutralising antibodies against this virus demonstrating the high variety of cross-reactive antibodies produced by this approach. These data illustrate the usefulness of this technology: Using a high diversity of similar proteins derived from multiple corona viruses fused to a nanoparticle leads to the production of antibodies that can protect from a wide range of different viruses and potentially even from future variants that have not yet emerged.
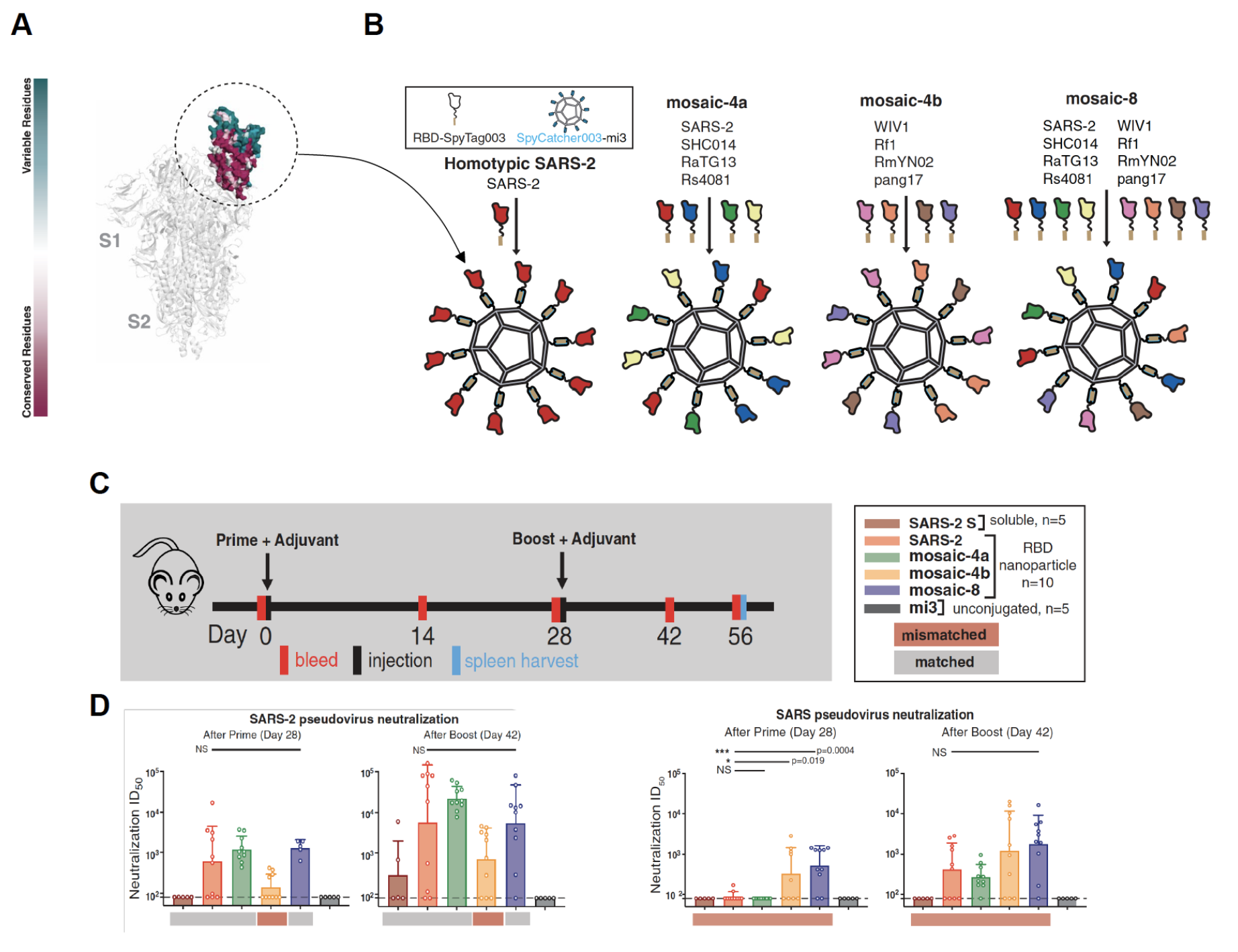
Figure 2: A Mosaic nanoparticle vaccine inducing cross-reactive antibody response.
A) Structure of SARS-CoV-2 S (Spike) trimer (PDB6VXX) with one RBD (dashed circle) in an “up” position. Colour scheme indicates conserved and variable regions between beta corona viruses. B) Spy-Tagged RBDs were attached to SpyCatcher003-mi3 to make a homotypic particle and three mosaic particles. There are 60 potential coupling sites on mi3; only 10 are shown for clarity C) Immunization schedule; adjuvant is AddaVax (Invivogen). D) Neutralisation data for serum IgGs for recognition of SARS2 pseudovirus (left) and SARS pseudovirus (right). Modified from Cohen et al., 2021.
The shape of things to come
New approaches are needed to finally contain SARS-CoV2. The prospect of a pan-corona vaccine is highly encouraging and might not only end the ongoing pandemic but also prevent future ones. The rapid development of new vaccine technologies and our improved understanding of the immune system response towards different virus variants and families will greatly help us prepare for the emergence of future variants and will potentially allow us to protect us not only from SARS-CoV2 but also other related virus families in the future.
References and resources:
Shinde, V., Bhikha, S., Hoosain, Z., Archary, M., Bhorat, Q., Fairlie, L., Lalloo, U., Masilela, M.S.L., Moodley, D., Hanley, S., et al. (2021). Efficacy of NVX-CoV2373 Covid-19 Vaccine against the B.1.351 Variant. New England Journal of Medicine 384, 1899–1909.
Bruun, T.U.J., Andersson, A.-M.C., Draper, S.J., and Howarth, M. (2018). Engineering a Rugged Nanoscaffold To Enhance Plug-and-Display Vaccination. ACS Nano 12, 8855–8866.
Saunders, K.O., Lee, E., Parks, R., Martinez, D.R., Li, D., Chen, H., Edwards, R.J., Gobeil, S., Barr, M., Mansouri, K., et al. (2021). Neutralizing antibody vaccine for pandemic and pre-emergent coronaviruses. Nature 594, 553–559.
Cohen, A.A., Gnanapragasam, P.N.P., Lee, Y.E., Hoffman, P.R., Ou, S., Kakutani, L.M., Keeffe, J.R., Wu, H.-J., Howarth, M., West, A.P., et al. (2021). Mosaic nanoparticles elicit cross-reactive immune responses to zoonotic coronaviruses in mice. Science 371, 735–741.